Products You May Like
How did everything begin? It’s a question that humans have pondered for thousands of years. Over the last century or so, science has homed in on an answer: the Big Bang.
This describes how the Universe was born in a cataclysmic explosion almost 14 billion years ago. In a tiny fraction of a second, the observable universe grew by the equivalent of a bacterium expanding to the size of the Milky Way. The early universe was extraordinarily hot and extremely dense. But how do we know this happened?
Let’s look first at the evidence. In 1929, the American astronomer Edwin Hubble discovered that distant galaxies are moving away from each other, leading to the realisation that the universe is expanding.
If we were to wind the clock back to the birth of the cosmos, the expansion would reverse and the galaxies would fall on top of each other 14 billion years ago. This age agrees nicely with the ages of the oldest astronomical objects we observe.
The idea was initially met with scepticism – and it was actually a sceptic, the English astronomer Fred Hoyle, who coined the name. Hoyle sarcastically dismissed the hypothesis as a ” Big Bang” during an interview with BBC radio on March 28 1949.
Then, in 1964, Arno Penzias and Robert Wilson detected a particular type of radiation that fills all of space. This became known as the cosmic microwave background (CMB) radiation. It is a kind of afterglow of the Big Bang explosion, released when the cosmos was a mere 380,000 years old.
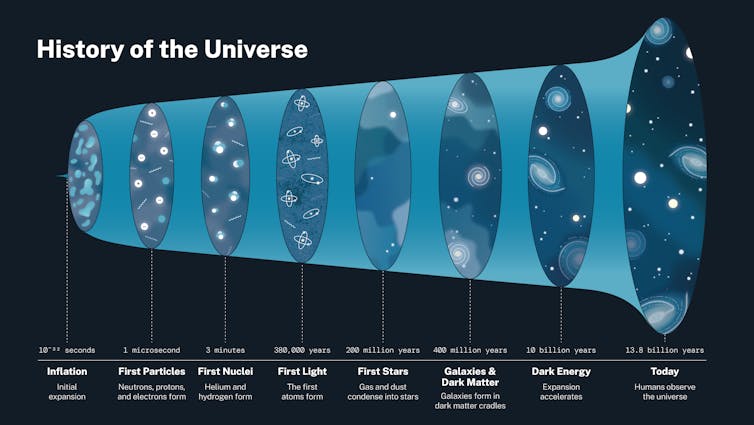
The CMB provides a window into the hot, dense conditions at the beginning of the universe. Penzias and Wilson were awarded the 1978 Nobel Prize in Physics for their discovery.
More recently, experiments at particle accelerators like the Large Hadron Collider (LHC) have shed light on conditions even closer to the time of the Big Bang. Our understanding of physics at these high energies suggests that, in the very first moments after the Big Bang, the four fundamental forces of physics that exist today were initially combined in a single force.
The present day four forces are gravity, electromagnetism, the strong nuclear force and the weak nuclear force. As the universe expanded and cooled down, a series of dramatic changes, called phase transitions (like the boiling or freezing of water), separated these forces.
Experiments at particle accelerators suggest that a few billionths of a second after the Big Bang, the latest of these phase transitions took place. This was the breakdown of electroweak unification, when electromagnetism and the weak nuclear force ceased to be combined. This is when all the matter in the Universe assumed its mass.
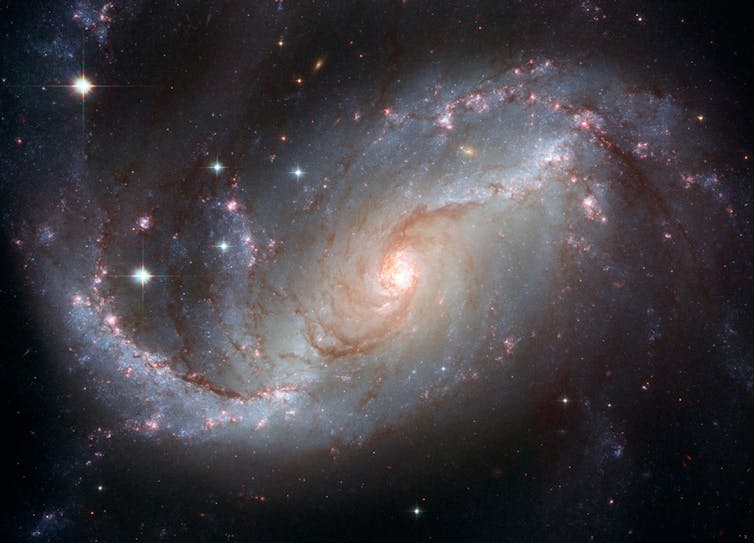
Moving on further in time, the universe is filled with a strange substance called quark-gluon plasma. As the name suggests, this “primordial soup” was made up of quarks and gluons. These are sub-atomic particles that are responsible for the strong nuclear force. Quark-gluon plasma was artificially generated in 2010 at the Brookhaven National Laboratory and in 2015 at the LHC.
Quarks and gluons have a strong attraction for one other and today are bound together as protons and neutrons, which in turn are the building blocks of atoms. However, in the hot and dense conditions of the early universe, they existed independently.
The quark-gluon plasma didn’t last long. Just a few millionths of a second after the Big Bang, as the universe expanded and cooled, quarks and gluons clumped together as protons and neutrons, the situation that persists today. This event is called quark confinement.
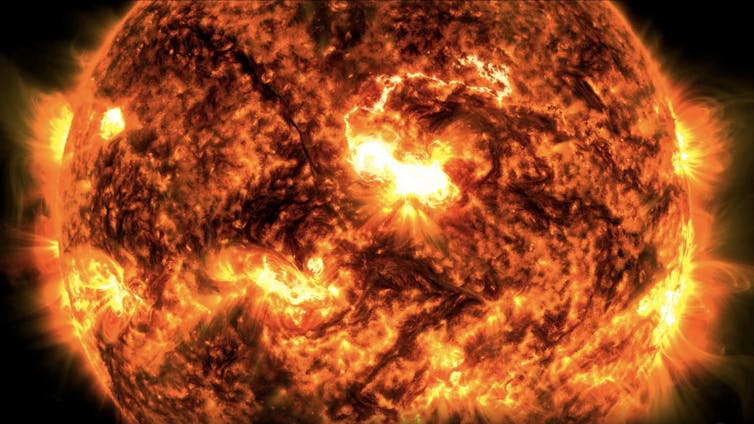
As the universe expanded and cooled still further, there were fewer high energy photons (particles of light) in the universe than there had previously been. This is a trigger for the process called Big Bang nucleosynthesis (BBN).
This is when the first atomic nuclei – the dense lumps of matter made of protons and neutrons and found at the centres of atoms – formed through nuclear fusion reactions, like those that power the Sun.
Back when there were more high energy photons in the universe, any atomic nuclei that formed would have been quickly destroyed by them (a process called photodisintegration). BBN ceased just a few minutes after the Big Bang, but its consequences are observable today.
Observations by astronomers have provided us with evidence for the primordial abundances of elements produced in these fusion reactions. The results closely agree with the theory of BBN. If we continued on, over nearly 14 billion years of time, we would reach the situation that exists today. But how close can we get to understanding what was happening near the moment of the Big Bang itself?
Scientists have no direct evidence for what came before the breakdown of electroweak unification (when electromagnetism and the weak nuclear force ceased to be combined). At such high energies and early times, we can only stare at the mystery of the Big Bang. So what does theory suggest?
When we go backwards in time through the history of the cosmos, the distances and volumes shrink, while the average energy density grows. At the Big Bang, distances and volumes drop to zero, all parts of the universe fall on top of each other and the energy density of the universe becomes infinite.
Our mathematical equations, which describe the evolution of space and the expansion of the cosmos, become infested by zeros and infinities and stop making sense.
We call this a singularity. Albert Einstein’s theory of general relativity describes how spacetime is shaped. Spacetime is a way of describing the three-dimensional geometry of the universe, blended with time. A curvature in spacetime gives rise to gravity.
But mathematics suggests there are places in the universe where the curvature of spacetime becomes unlimited. These locations are known as singularities. One such example can be found at the centre of a black hole. At these places, the theory of general relativity breaks down.
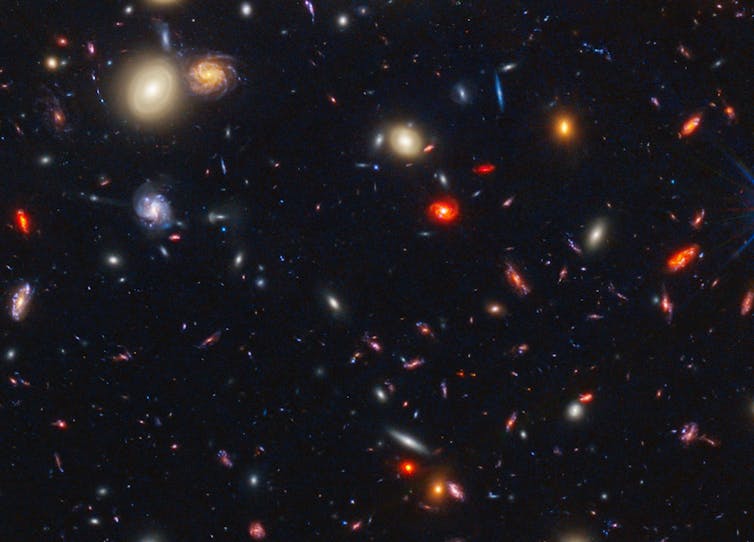
From 1965 to 1966, the British theoretical physicists Stephen Hawking and Roger Penrose presented a number of mathematical theorems demonstrating that the spacetime of an expanding universe must end at a singularity in the past: the Big Bang singularity.
Penrose received the Nobel Prize in 2020. Hawking passed away in 2018 and Nobel Prizes are not awarded posthumously. Space and time appear at the Big Bang singularity, so questions of what happens “before” the Big Bang are not well defined. As far as science can tell, there is no before; the Big Bang is the onset of time.
However, nature is not accurately described by general relativity alone, even though the latter has been around for more than 100 years and has not been disproven. General relativity cannot describe atoms, nuclear fusion or radioactivity. These phenomena are instead addressed by quantum theory.
Theories from “classical” physics, such as relativity, are deterministic. This means that certain initial conditions have a definite outcome and are therefore absolutely predictive. Quantum theory, on the other hand, is probabilistic. This means that certain initial conditions in the universe can have multiple outcomes.
Quantum theory is somewhat predictive, but in a probabilistic way. Outcomes are assigned a probability of existing. If the mathematical distribution of probabilities is sharply peaked at a certain outcome, then the situation is well described by a “classical” theory such as general relativity.
But not all systems are like this. In some systems, for example atoms, the probability distribution is spread out and a classical description does not apply.
What about gravity? In the vast majority of cases, gravity is well described by classical physics. Classical spacetime is smooth.
However, when curvature becomes extreme, near a singularity, then the quantum nature of gravity cannot be ignored. Here, spacetime is no longer smooth, but gnarly, similar to a carpet which looks smooth from afar but up-close is full of fibres and threads.
Thus, near the Big Bang singularity, the structure of spacetime ceases to be smooth. Mathematical theorems suggest that spacetime becomes overwhelmed by “gnarly” features: hooks, loops and bubbles. This rapidly fluctuating situation is called spacetime foam.
In spacetime foam, causality does not apply, because there are closed loops in spacetime where the future of an event is also its past (so its outcome can also be its cause).
The probabilistic nature of quantum theory suggests that, when the probability distribution is evenly spread out, all outcomes are equally possible and the comfortable notion of causality we associate with a classical understanding of physics is lost.
Therefore, if we go back in time, just before we encounter the Big Bang singularity, we find ourselves entering an epoch where the quantum effects of gravity are dominant and causality does not apply. This is called the Planck epoch.
Time ceases to be linear, going from the past to the future, and instead becomes wrapped, chaotic and random. This means the question “why did the Big Bang occur?” has no meaning, because outside causality, events do not need a cause to take place.
In order to understand how physics works at a singularity like the Big Bang, we need a theory for how gravity behaves according to quantum theory. Unfortunately, we do not have one. There are a number of efforts on this front like loop quantum gravity and string theory, with its various incarnations.
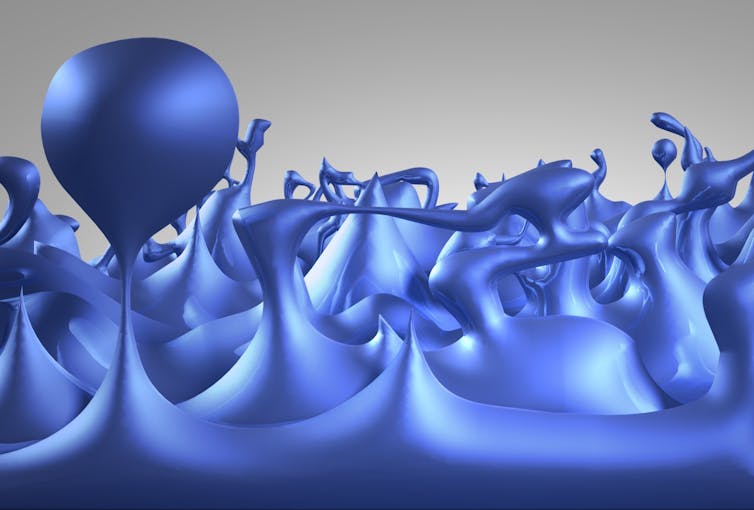
However, these efforts are at best incomplete, because the problem is notoriously difficult. This means that spacetime foam has a totemic, powerful mystique, much like the ancient Chaos of Hesiod which the Greeks believed existed in the beginning.
So how did our expanding and largely classical universe ever escape from spacetime foam? This brings us to cosmic inflation. The latter is defined as a period of accelerated expansion in the early universe. It was first introduced by the Russian theoretical physicist Alexei Starobinsky in 1980 and in parallel, that same year, by the American physicist Alan Guth, who coined the name.
Inflation makes the universe large and uniform, according to observations. It also forces the universe to be spatially flat, which is an otherwise unstable situation, but which has also been confirmed by observations.
Moreover, inflation provides a natural mechanism to generate the primordial irregularities in the density of the universe that are essential for structures such as galaxies and galaxy clusters to form.
Theory vindicated
Precision observations of the cosmic microwave background in recent decades have spectacularly confirmed the predictions of inflation. We also know that the universe can indeed undergo accelerated expansion, because in the last few billion years it started doing it again.
What does this have to do with spacetime foam? Well, it turns out that, if the conditions for inflation arise (by chance) in a patch of fluctuating spacetime, as can occur with spacetime foam, then this region inflates and starts conforming to classical physics.
According to an idea first proposed by the Russian-American physicist Andrei Linde, inflation is a natural – and perhaps inevitable – consequence of chaotic initial conditions in the early universe.
The point is that our classical universe could have emerged from chaotic conditions, like those in spacetime foam, by experiencing an initial boost of inflation. This would have set off the expansion of the universe. In fact, the observations by astronomers of the CMB suggest that the initial boost is explosive, since the expansion is exponential during inflation.
In March 20 of 2014, Alan Guth explained it succinctly: “I usually describe inflation as a theory of the ‘bang’ of the Big Bang: It describes the propulsion mechanism that we call the Big Bang.”
So, there you have it. The 14 billion year story of our universe begins with a cataclysmic explosion everywhere in space, which we call the Big Bang. That much is beyond reasonable doubt.
This explosion is really a period of explosive expansion, which we call cosmic inflation. What happens before inflation, though? Is it a spacetime singularity, is it spacetime foam? The answer is largely unknown.
In fact, it might even be unknowable, because there is a mathematical theorem which forbids us from accessing information about the onset of inflation, much like the one that prevents us from knowing about the interiors of black holes. So, from our point of view, cosmic inflation is the Big Bang, the explosion that started it all.
Konstantinos Dimopoulos, Professor in Particle Cosmology, Lancaster University
This article is republished from The Conversation under a Creative Commons license. Read the original article.