Products You May Like
The Sun’s rotation is, in a word, bizarre.
You’d sort of expect every latitude of its surface to spin at more or less the same rate, but no. If you could stand on the Sun’s equator, for example, it would take you approximately 24 Earth days to undergo a full rotation. If you stood on either of the poles, it would take around 34 days to return to your original orientation.
This is known as differential rotation, and it has puzzled scientists for a long time.
It’s only become more puzzling as we probe deeper and deeper into the solar interior. Helioseismological observations reveal that the phenomenon is not restricted to the upper atmosphere – it extends down some 200,000 kilometers (124,000 miles), through the entire solar convection zone.
Now, a team led by solar physicist Yuto Bekki of the Max Planck Institute for Solar System Research (MPS) have figured out a clue. The differential rotation seems to be reined in by long-period oscillations of sound waves in the convection zone that can be detected on the surface as swirling motions around the poles.
frameborder=”0″ allow=”accelerometer; autoplay; clipboard-write; encrypted-media; gyroscope; picture-in-picture; web-share” referrerpolicy=”strict-origin-when-cross-origin” allowfullscreen>
The Sun is constantly ‘humming’. The visible surface layer known as the photosphere is buzzing with millions of acoustic oscillation modes rising and falling in periods of around five minutes.
We’ve known about these modes for a while, but just a few years ago a team of researchers led by MPS director Laurent Gizon found a new type of acoustic oscillation. Using several years’ worth of solar observation data, they found a global oscillation mode with a far longer 27-day period.
And there was something else. These giant sound waves rippling through the Sun seemed to be linked, somehow, to the solar differential rotation.
Back in 2021, when the original finding was published, the researchers thought that the long-term oscillation modes relied on the differential rotation. But on closer investigation, Bekki and his colleagues found that the relationship goes both ways. The differential rotation is curtailed by the giant sound waves.
To investigate the relationship between the two, he and his colleagues conducted three-dimensional numerical simulations, exploring the effects of the oscillations. The researchers found that the modes at high latitudes – those that circle the poles – have a profound effect on the Sun’s behavior by transporting heat from the poles to the equatorial region.
Because the poles are warmer than the equator, this heat transport limits the temperature difference between the two latitudinal regions. It means that the contrast between the poles and the equator cannot exceed 7 Kelvin (7 degrees Celsius, or 12.6 degrees Fahrenheit).
Although this difference is tiny when talking about a ball of hot plasma that roils at thousands of degrees, it’s this temperature range that ultimately controls the differential rotation.
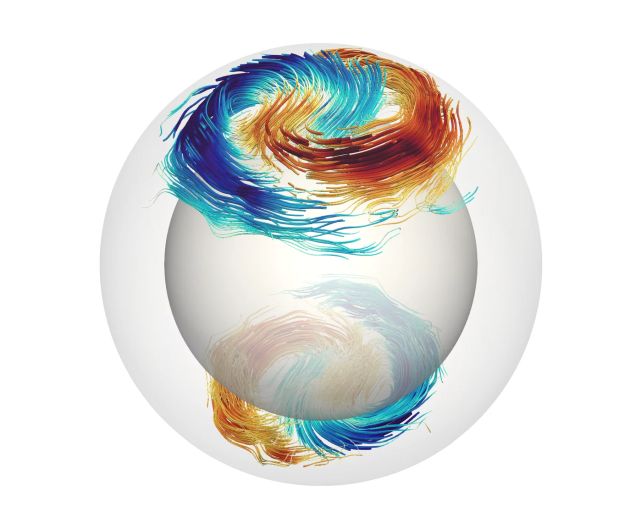
“This very small temperature difference between the poles and the equator controls the angular momentum balance in the Sun and thus is an important feedback mechanism for the Sun’s global dynamics,” Gizon explains.
Although the processes are different in some ways, it’s similar to the way atmospheric instabilities can produce giant cyclonic storms on Earth. And while there’s still quite a mystery to be solved, the link between the processes could help us get there. The high-latitude oscillation modes play a significant role in guiding the Sun’s differential rotation. And perhaps the same dynamics are at play on other stars.
The Sun is a big ol’ hinky ball of flame in the sky, full of mysteries and enigmas. Little by little, we’re getting closer to resolving them.
The research has been published in Science Advances.