Products You May Like
Ions inside a compact fusion reactor barely a meter (less than 3 feet) across have been heated to the magic figure of 100 million degrees Celsius (some 180 million degrees Fahrenheit) for the first time in a monumental step towards making nuclear fusion energy a practical reality.
Researchers from Tokamak Energy Ltd in the UK, Princeton and Oak Ridge National Laboratory in the US, and the Institute for Energy and Climate Research in Germany, achieved the record on a device at a spherical tokamak (ST), which unlike the more circular ‘donut shaped’ paths heated fuel takes in larger reactors, confines plasma in an ‘cored-apple shaped‘ swirl intended to improve stability and practicality of power generation.
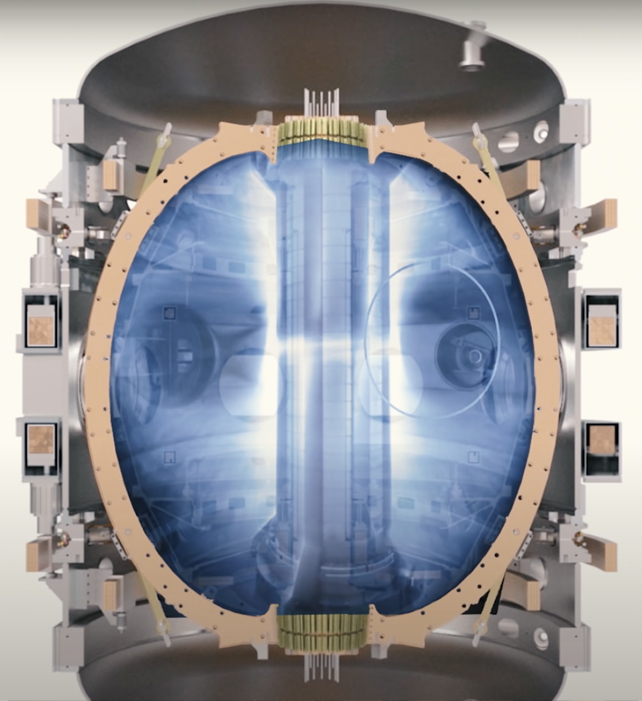
Nuclear fusion replicates fundamental processes in the core of our own Sun and stars like it, squeezing energy from the melding of smaller elements into larger ones. If we can get it right – and it’s quite a big if – it means a virtually inexhaustible source of clean energy, with no harmful emissions attached.
Where stars have huge amounts of gravity at their disposal to fuse elements and release energy, we’re forced to rely on heat. A lot of heat, in fact, equivalent to several times hotter than the Sun’s core.
Cooking the atomic ingredients, or ions, to least 100 million degrees Celsius (essentially anything over 100 million degrees Kelvin, or 8.6 kiloelectronvolts in energy terms) is crucial for achieving the right pressures.
“Ion temperatures in excess of 5 keV [kiloelectronvolts ] have not previously been reached in any ST and have only been obtained in much larger devices with substantially more plasma heating power,” write the researchers in their published paper.
In this case a spherical tokamak called ST40 was used. Putting aside the machinery necessary for it to operate safely, the reactor itself is a mere 0.8 meters across, a mere fraction of the larger tokamaks that can stretch several meters in diameter.
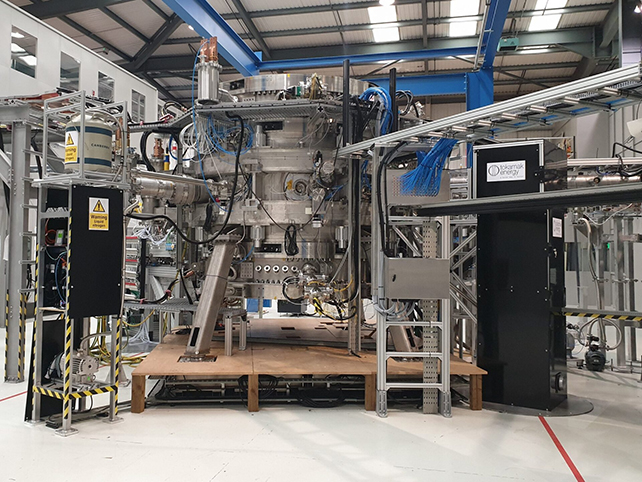
Compared with larger fusion reactors, these smaller devices are cheaper to build, and potentially more efficient and more stable – all advantages if you want to make a technology commercially viable.
A number of optimizations were deployed by the researchers to reach the new temperature record, including the use of the ST itself, and the way the plasma was prepared in terms of how it was heated and its electron density.
Some techniques were borrowed from ‘supershot’ experiments carried out in the 1990s in the Tokamak Fusion Test Reactor, which is much larger than the ST40. Essentially, the approach involved a lot of heat being applied in a very short space of time.
Another optimization trick applied by the scientists was to heat up the positively charged ions more than the negatively charged electrons inside the plasma. Known as a hot-ion mode, it helps to increase the number of reactions and the tokamak performance.
“These temperatures were achieved in hot-ion mode scenarios, where the ion temperature exceeds that of the electrons, typically by a factor of two or greater,” write the researchers.
While this breakthrough and others like it are certainly exciting, nuclear fusion is still very much in a test phase with multiple hurdles to yet clear before it could be considered as a practical source of power. Not everyone believes that nuclear fusion power production is ultimately going to be possible, considering the technical challenges involved.
Those challenges are highlighted here too: the top temperature was reached for a mere 150 milliseconds. A fine achievement in the lab, but not much time to practically contribute anything to the energy grid.
Still, each discovery brings us closer to the ultimate goal – and this one is particularly notable, considering that spherical tokamaks are one of the most promising options for creating nuclear fusion reactions in a way that the necessary energy and economic equations end up making sense.
“These results demonstrate for the first time that ion temperatures relevant for commercial magnetic confinement fusion can be obtained in a compact high-field ST and bode well for fusion power plants based on the high-field ST,” write the researchers.
The research has been published in Nuclear Fusion.