Products You May Like
The Standard Model of particle physics is our current best-guess on what the blue-prints for matter looks like. Of all of its predictions, none are as precise as the magnetic moment of the electron.
Not only is it precisely predicted, it’s among the most accurately measured of any particle’s properties. And while these two values are close, they don’t overlap entirely, providing tantalizing hints of new physics.
Getting closer to the exact value of the electron magnetic moment – simply put, how strongly an electron behaves like a tiny magnet – might one day unlock a greater understanding of the building blocks of physics and how they interact.
Now physicists from Harvard University and Northwestern University have pushed the limits on that accuracy further still. Their recent experiments produced a value that’s precise to 0.13 out of 1 trillion.
“The new value is 2.2 times more precise than, and consistent with, the one that stood for 14 years,” write the researchers in their published paper.
“Our determination and the Standard Model calculation are precise enough for a test that is 10 times more precise.”
To get the new electron magnetic moment value, the research team suspended a single electron in a highly controlled chamber known as a Penning trap.
After chilling the chamber close to absolute zero, the team was able to use a magnetic field to measure the electron’s “quantum jumps” between energy levels, without interfering with its quantum state and spoiling the observations.
The Standard Model’s equations provide a way to calculate something called the fine-structure constant. Roughly equivalent to 1/137, it is fundamental to the electromagnetic force that binds atoms, making it a pretty big deal in physics.
These same equations predict the electron magnetic moment to such an impressive level of precision that its measure in the lab has become a defining test of the Standard Model’s ability to reflect reality.
For some time, measurements of the electron magnetic moment have remained slightly larger than what the Standard Model predicts for a charged, point-like particle, producing an alluring anomaly begging to be resolved.
These new results have an error margin that’s ten times smaller than this discrepancy, strongly hinting at unknown physics.
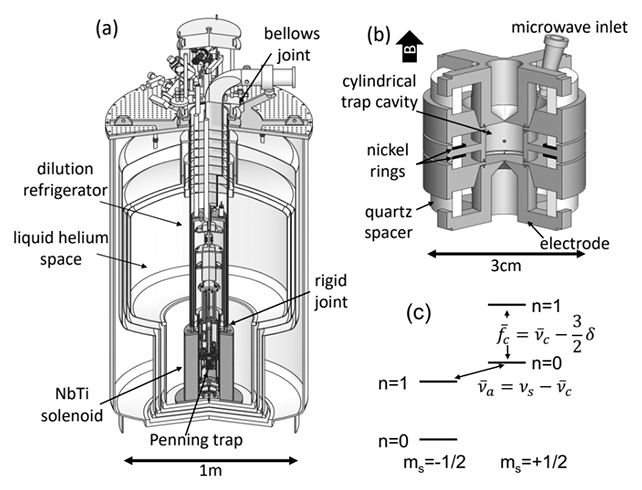
Fine tuning both predictions and experimental results could deliver values that hint at the existence of new particles or types of interactions we don’t yet know about.
The researchers already have ideas for how to improve the measurement, represented as μ/μB (the comparison of a value to the Bohr magneton), even further. It gets us ever closer to finally completing the Standard Model of particle physics.
“Much larger improvements in the precision of μ/μB now seem feasible given the demonstration of more stable apparatus, improved statistics, and better understood uncertainties,” write the researchers.
The research has been published in Physical Review Letters.